Talk Abstracts
Materials Innovation and Interfacial Science for Better Batteries
Y. Shirley Meng
Laboratory for Energy Storage & Conversion, The University of Chicago, IL, USA; Argonne Collaborative Center for Energy Storage Science (ACCESS), IL, USA
Batteries have become increasingly important for our society as they are the key enabling technology for energy transition. In the next couple of decade, we expect to see batteries that last longer, more powerful and safer. Materials science play a more critical role than most people realize. For example, Lithium (Li) metal has been considered as an ideal anode for high-energy rechargeable Li batteries while Li nucleation and growth at the nano scale remains mysterious as to achieving reversible stripping and deposition. A few decades of research have been dedicated to this topic and we have seen breakthroughs in novel electrolytes in the last few years, where the efficiency of lithium deposition is exceeding 99.5%. Cryogenic-transmission electron microscopy (Cryo-TEM/Cryo-FIB) was used to reveal the evolving nanostructure of Li deposits at various transient states in the nucleation and growth process, in which a disorder-order phase transition was observed as a function of current density and deposition time. More importantly, the complementary techniques such as titration gas chromatography (TGC) reveals the important insights about the phase fraction of solid electrolyte interphases (SEI) and electrochemical deposited Li (EDLi). While cryo-EM has made significant contributions to enabling lithium metal anodes for batteries, its applications in the area of solid state electrolytes, metal-free sulfur cathodes remain in its infancy. In this talk, I will discuss a few new perspectives about how materials innovation and interfacial science can accelerate the innovation of next generation energy storage materials and architectures.
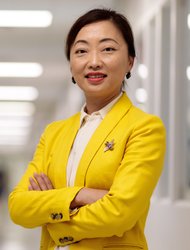
Dr. Y. Shirley Meng is a Professor at the Pritzker School of Molecular Engineering at the University of Chicago. She serves as the Chief Scientist of the Argonne Collaborative Center for Energy Storage Science (ACCESS) Argonne National Laboratory. Dr. Meng is the principal investigator of the research group - Laboratory for Energy Storage and Conversion (LESC), that was established at University of California San Diego since 2009. She held the Zable Chair Professor in Energy Technologies at University of California San Diego (UCSD) from 2017-2022. Dr. Meng received several prestigious awards, including ECS Battery Division Research Award (2023), the C3E technology and innovation award (2022), the Faraday Medal of Royal Chemistry Society (2020), International Battery Association IBA Research Award (2019), Blavatnik Awards for Young Scientists Finalist (2018), C.W. Tobias Young Investigator Award of the Electrochemical Society (2016) and NSF CAREER Award (2011). Dr. Meng is elected Fellow of Electrochemical Society (FECS), Fellow of Materials Research Society (FMRS) and Fellow of American Association for the Advancement of Science (AAAS). She is the author and co-author of more than 300 peer-reviewed journal articles, two book chapters and eight issued patents. She is the Editor-in-Chief for Materials Research Society MRS Energy & Sustainability. Dr. Meng received her Ph.D. in Advance Materials for Micro & Nano Systems from the Singapore-MIT Alliance in 2005. She received her bachelor's degree in Materials Science with first class honor from Nanyang Technological University of Singapore in 2000.
Fabrication of Utility-Scale Quantum Computers
John Martinis
University of California, Santa Barbara and Qolab
The next important milestone for quantum computing is moving past quantum supremacy to quantum utility - solving useful computations faster than classical computers. Although there are two schools of thought for accomplishing this, via NISQ algorithms or error correction, both require significant improvements in qubit quality that lowers errors to the 10^-3 to 10^-4 range. For superconducting qubits, I will discuss how this goal can be achieved using the combination of fast tunable couplers and improvements in coherence time. I will also describe a vision of how superconducting qubits should be fabricated in the upcoming years, moving beyond academic-style processing, to improve materials and yield.
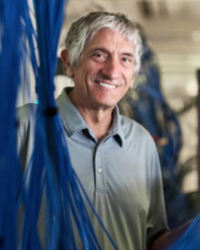
Dr. John Martinis is professor of physics at the University of California, Santa Barbara where he explores the physics of superconducting devices, focusing on achieving very low noise and high sensitivity performance. His primary interest at present is to build a quantum computer based on Josephson junction quantum bits. Martinis did pioneering experiments On superconducting qubits for his PhD thesis at University of California, Berkeley.
He was a postdoctoral researcher at Commissariat à l'Energie Atomique in Saclay, France, and then went onto the Electromagnetic Technology division at NIST in Boulder, CO, where he worked on superconducting quantum interference devices (SQUIDs) amplifiers. In 2004 he joined the University of California, Santa Barbara as a professor in physics. From 2014 to 2020 Martinis and his group worked with Google to build a useful quantum computer, culminating in a quantum supremacy experiment in 2019.
He has worked on a variety of low temperature device physics during his career, focusing on quantum computation since the late 1990s. He was awarded the London Prize in low temperature physics in 2014 for his work in this field. He was awarded the John Stewart Bell prize in 2021.
Innovation Accelerated: Disruptive Approaches for the Next Era of Semiconductor Manufacturing
Tim Archer
President and CEO, Lam Research
We are in the midst of a technological revolution enabled by decades of innovation in the semiconductor industry. Today's most advanced semiconductor products enable technologies such as artificial intelligence, autonomous vehicles, and ubiquitous connectivity. While the world celebrates advances like large language models and spatial computing, the growing cost, complexity and environmental impact of semiconductor production at the nanoscale threatens to slow the pace of innovation and adoption. By adding virtual twins, advanced simulation, and artificial intelligence into the multi-disciplinary toolbox of semiconductor process engineers, we are opening pathways to sustainably address the industry's most difficult challenges and develop the future semiconductor workforce on a global scale.
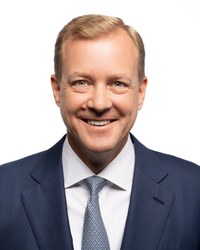
Timothy M. Archer is president and chief executive officer at Lam Research. He joined the company in 2012 following its acquisition of Novellus Systems, Inc. ("Novellus") when he began serving as Lam's executive vice president and chief operating officer. He was later promoted to president and chief operating officer, and then president and chief executive officer in December 2018.
Tim currently serves on the International Board of Directors for SEMI, the global industry association representing the electronics manufacturing and design supply chain. From 2020 to 2022, he served as chairman of the board for the National Consortium for Graduate Engineering Degrees for Minorities (GEM): a nonprofit organization in the U.S. that is dedicated to increasing the participation of underrepresented groups in engineering and science at the master's and doctoral levels.
Prior to joining Lam, Tim had an 18-year tenure at Novellus where he served as the company's COO, and held other executive positions supporting worldwide sales, marketing, customer satisfaction, and product and business development.
Archer completed the Program for Management Development at the Harvard Graduate School of Business and earned a B.S. degree in applied physics from the California Institute of Technology.
Keynote Talk by Dr. Steven Chu
William R. Kenan Jr. Professor, Professor of Molecular and Cellular Physiology and of Energy Science and Engineering at Stanford University
Throughout human history, the synthesis of new materials has enabled multiple industrial and agricultural revolutions that have profoundly transformed the world. However, the unintended consequence of these revolutions is that the greenhouse gas emissions are changing Earth's climate. Most of our energy sources, materials, and chemicals are fossil-fuel based or demand process heat. The challenges how to provide clean energy, water, air, food in a world of over 8 billion people and likely to grow to 11 billion by 2100 are formidable. Following a brief review of how materials transformed our lives, I will discuss how new materials can help us achieve net-zero greenhouse emissions.
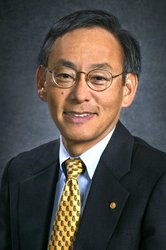
Steven Chu is the William R. Kenan, Jr. Professor of Physics, of Molecular and Cellular Physiology, and of Energy Science and Engineering at Stanford University. He received an A.B. degree in mathematics and a B.S. degree in physics from the University of Rochester, and a Ph.D. in physics from the University of California, Berkeley. After a postdoctoral fellowship at Berkeley, he was at Bell Labs as a member of the technical staff in 1978 and then department head in 1983.
From January 2009 to April, 2013, Dr. Chu served as U.S. Secretary of Energy under President Barack Obama. During his tenure, he began several initiatives, including ARPA-E (Advanced Research Projects Agency – Energy), the Energy Innovation Hubs, and the Clean Energy Ministerial meetings. As the first scientist Cabinet member, Chu recruited dozens outstanding scientists and engineers to the Department of Energy, and was personally tasked by President Obama to help stop the BP Oil leak.
From 2004-2009, he was the director of the Lawrence Berkeley National Laboratory and Professor of Physics and of Molecular and Cell Biology at the University of California Berkeley. Prior to those positions, he was the Theodore and Francis Geballe Professor of Physics and Applied Physics at Stanford University. During this time, he helped start Bio-X, a multi-disciplinary initiative combining the physical and biological sciences with engineering and medicine.
His contributions include the introduction of laser cooling and optical trapping of atoms and particles, atomic fountain clocks and atom interferometers, the optical tweezers of biomolecules, and single molecule FRET of biomolecules tethered to surfaces. His current research is in biophysics, molecular and cellular physiology, medical imaging, nanoparticle synthesis and battery research. He has received many awards, including the 1997 Nobel Prize for laser cooling and optical trapping of atoms. He is a member of the National Academy of Sciences, the American Philosophical Society, the American Academy of Arts and Sciences, National Academy of Inventors, and a foreign member of the Royal Society, the Royal Academy of Engineering, the Chinese Academy of Sciences, the Academia Sinica, the Korean Academy of Sciences and Technology and the Pontifical Academy of Sciences.
He received an A.B. degree in mathematics, and a B.S. degree in physics from the University of Rochester, and a Ph.D. in physics from the University of California, Berkeley, and 35 honorary degrees.