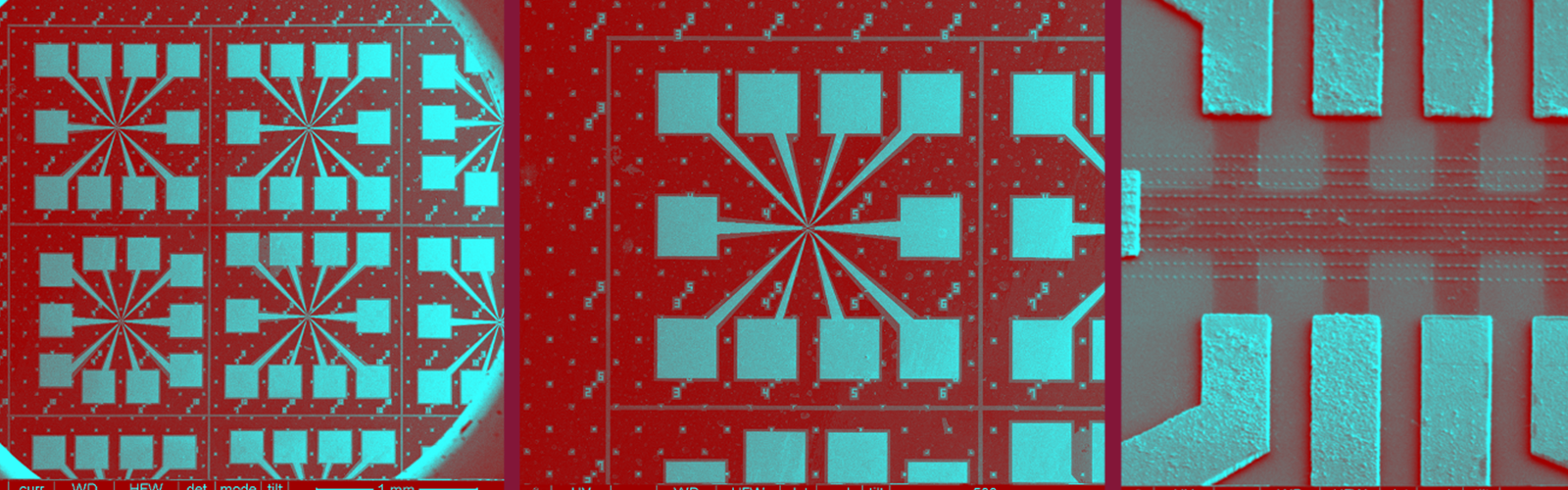
Quantum Straintronics
Emerging quantum and topological phenomena from nano-devices based on 2D van der Waals materials and heterostructures
Two-dimensional (2D) van der Waals (vdW) materials, such as graphene, hexagonal boron nitride (h-BN) and transition metal dichalcogenides (TMDs), are quantum materials that exhibit a rich variety of novel properties that can be further tailored to achieve new functionalities and emerging quantum and topological phenomena by such approaches as nanoscale strain engineering and nanoscale plasmonic metasurface-controlled light-matter interaction. Nai-Chang Yeh, Thomas W. Hogan Professor of Physics, and her research group utilize the nanofabrication facilities at the KNI to develop various novel nano-devices based on heterostructures of 2D-vdW materials to explore their electrical, optical, and optoelectronic properties.
For example, the Yeh group has established a new paradigm of quantum straintronics for controlling the 2D electronic correlation and bandstructures of single layer (SL) graphene via nanoscale engineering of the underlying SiO2/Si substrate, which leads to periodic graphene wrinkles with controlled patterns and strengths of strain that induces spatially varying giant pseudo-magnetic fields (PMF, see Figure 1) and fundamentally alters the electronic properties of monolayer graphene from valley-degenerate massless Dirac spectrum to valley-splitting flat band correlated electrons.[1,2] Emerging quantum phenomena from the valley-Hall transistors fabricated from such strained SL-graphene (see Figure 2 for exemplifying devices) have been discovered.
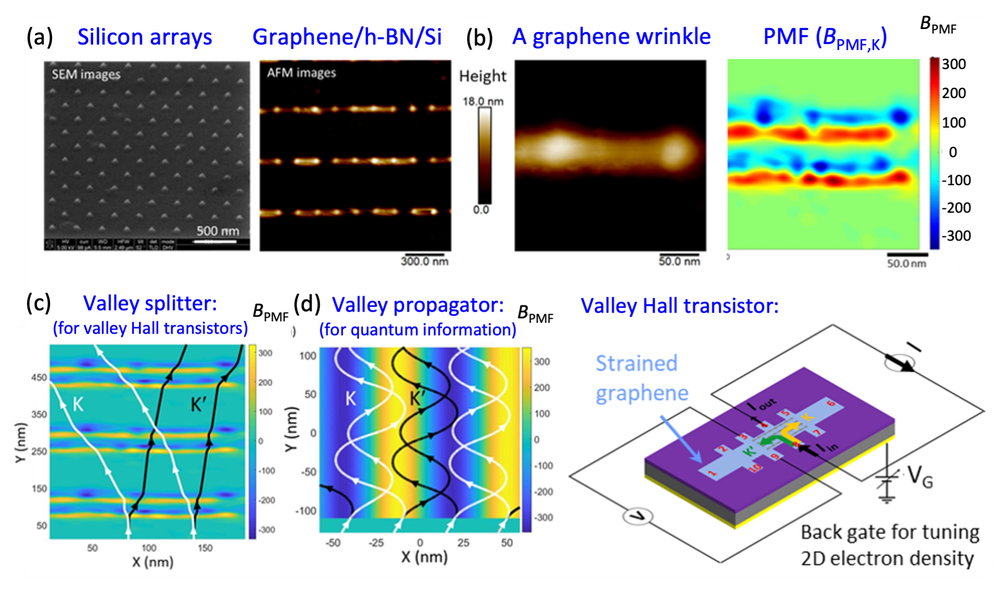
Figure 1: Nanoscale strain engineering of SL-graphene by fabrication of periodic arrays of nano-cones on SiO2/Si substrates.[1,2] (a) Left: SEM image of rectangular arrays of cone-shaped nanostructures fabricated on SiO2/Si. Right: AFM image of three parallel graphene wrinkles induced by the underlying nano-cone arrays shown in the left. (b) Left: AFM image of a graphene wrinkle. Right: The corresponding PMF for the K-valley as derived from the strain distribution in the left panel.[1] Semi-classical analyses[1] suggest that these parallel wrinkles in graphene behave like (c) a valley splitter for valley Hall transistors, or (d) as a valley propagator that collimates the propagation of different valleys K and K¢ along separate channels without mixing. (e) Schematic of a valley Hall transistor based on strained graphene wrinkles.
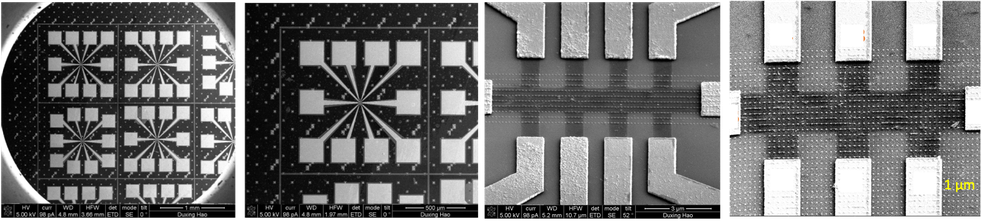
Figure 2: SEM images of different valley Hall transistors fabricated on the same piece of strained SL-graphene by e-beam lithography. The scale bars for the SEM images from left to right are 1 mm, 500 μm, 3 μm and 1 μm.
The Yeh group is also developing novel topological photonics and optoelectronics by applying optical and/or plasmonic vortices of different topological charges to 2D-vdW materials.[3,4] As an example (Figure 3), plasmonic Archimedes spiral (PAS) gold nanostructures with proper designs for the number of turns and the pitches are fabricated on top of a SL semiconducting TMD (e.g., WS2), the latter has been transferred to a substrate consisting of thin layers of SiO2 (60 nm) and Au mirror (150 nm) on Si. The PAS/SL-TMD heterostructure on SiO2/Au/Si thus form a metal-dielectric-metal (MDM) nanocavity to enhance the interaction of light with the SL-TMD sample.[3] The photoluminescence (PL) signals from the SL-TMD under the excitation of righthanded (RH) circularly polarized light (CPL) is found to be dramatically enhanced near the central region of the PAS/SL-TMD heterostructures by the presence of RH-PAS, whereas the excitation of lefthanded (LH) CPL does not lead to enhanced PL signals from the heterostructures of RH-PAS/SL-TMD.[3] The enhancement of the PL signal is the result of light focusing effects of the PAS and the optical selection rules associated with the topological charges of the excitation light and the PAS.[3] Similar approaches involving twisted light (i.e., photons with finite orbital angular momenta ℓ = ±1, ±2, ±3…)[4] interacting with the PAS/SL-TMD heterostructures for PAS nanostructures of varying topological charges may be devised to yield topological photonic and optoelectronic devices for high-fidelity optical communication.
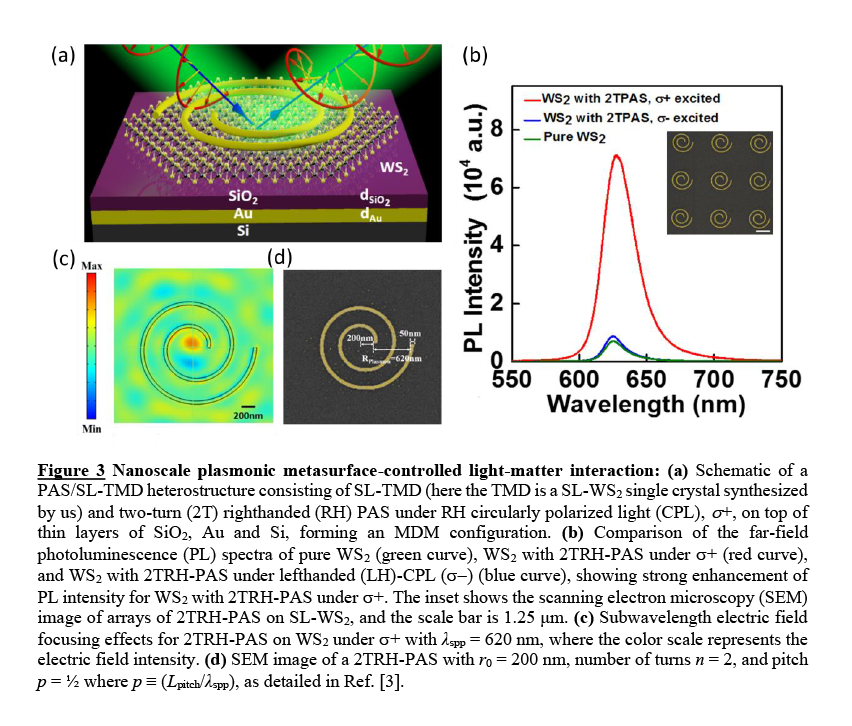